As the title hints, I have been here before. The SN1 solvolysis mechanism of t-butyl chloride was central to the flourishing of physical organic chemistry from the 1920s onwards, and it appears early on in most introductory lecture courses and text books. There we teach that it is a two-stage mechanism. Firstly the C-Cl bond heterolyses to form a stable tertiary carbocation intermediate, which in a second stage reacts with nucleophile (water) to form e.g. t-butanol. This is contrasted with the SN2 mechanism, where these two stages are conflated into a single concerted process, involving no intermediates. Here I explore an intrinsic reaction coordinate for the hydrolysis of t-butyl chloride which attempts to tease out whether this simple picture is realistic.
The basic model comprises t-butyl chloride and 16 water molecules. These are subjected to a wB97XD/6-311G(d,p) calculation with a continuum water solvent field applied throughout. The functional is different from the one I used last time, since I wanted one that included dispersion attractions. The basis set is also better.
- At IRC -5, we see the first stage of the mechanism, the cleavage of the C-Cl bond. Note how the methyl flag waves at this point.
- At IRC 0.0 we have the transition state, at which point the gradients of the energy are precisely zero.
- At IRC +5, we have a very slight dip/inflexion point in the potential, but the gradients do not actually go to zero. This is the point that would correspond to the formation of a carbocation. The SN1 mechanism proper would require a formal intermediate here, with zero gradients.
- At IRC ~+15, we see a new phenomenon, the attack of a water molecule on the “almost” carbocation, reflecting in fact an SN2 mechanism.
- At IRC ~+20, we see a slight blip, which in fact is reorganisation of the hydrogen bonds of the surrounding water molecules, accompanying the formation of an entirely ionic chloride.
- All these processes are animated in the diagram below, where you can see other features:
- Note the methyl rotation just after the Cl has started leaving, and another when the C-O bond formation is completing.
- Note the hydrogen bond reorganisation near the end.
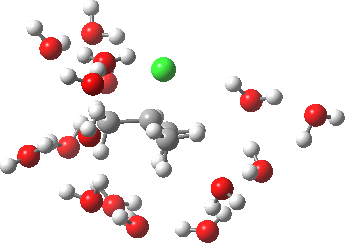
(anharmonic) IRC for hydrolysis of t-butyl chloride. Click to see the (harmonic) transition normal mode.
As I noted in my previous post on the topic, there are other complexities, involving potential proton transfers amongst the water molecules which is not reflected here. As is not unusual in science, sometimes the most apparently simple processes turn out to have hidden complexities.
Tags: Historical, IRC, SN1, SN2, Tutorial material
[…] derivative in the form of the gradient norm) flattens out. This reminds of what happened with the SN1 solvolysis of t-butyl chloride. However it does not quite go to zero. There is still the dynamic momentum for one more […]
[…] so-called E2 elimination mechanism is another one of those mainstays of organic chemistry. It is important because it introduces the principle […]
[…] to instead being a vinyl carbocation intermediate. Similar behaviour was computed for the purported Sn1 solvolysis of tert-butyl chloride, in which the tertiary carbocation is not an actual intermediate on the reaction path but is […]
[…] good example of such a species is the intermediate carbocation in the solvolysis of t-butyl chloride. This too is […]
[…] for R1=R2=R3 shows a qualitative difference, in resembling the mechanism for Sn1 solvolysis of t-butyl chloride in water. In this case the bond O-C bond labelled 2.3 is cleaving, whilst the C-O bond labelled 3.1 […]
Great set of blogs, Henry! But I missed seeing the typical Winstein version of solvolysis via conteact and then solvent-separated ions pairs. Don’t the latter exist, despite Winstein’s evidence? Even for more than one kind of them?
A profound question Paul. The solvent separated ion-pairs probably need time to reorganise. I think a more complete analysis of this apparently simple chemistry would have to allow the time-dependent dynamic diffusion of water molecules. At the moment, the diffusion is determined purely by the potential energy surface. Certainly, there was a phase where the solvent waters reorganised themselves, but that phase should probably be modelled using molecular dynamics rather than just potential energies.
Add to the mix the possibility of proton transfers between waters and the departing chloride, and the system starts to get very complex.
What we can say is that ONE trajectory for this system involves a concerted but asynchronous SN2 type reaction, where the chloride leaves in the first phase and the water quenches the hidden carbocation in the second. There are probably other trajectories where a water diffuses into the space between the cation and the chloride to produce a solvent-separated ion pair. Dynamics would tell us what the stochastic distribution of these trajectories is.
Henry, Your writings in #2 suggest the t-butyl “racemization” as arising from a “water-water exchange” after initial water displacement of chloride. Collapse of a solvent-separated ion pair would give retention (along witn the backside displacement giving inversion).
Have you looked for the solvent-separated ion pair by starting with an appropriate model geometry?
There is also the matter of internal return, shown by C. Wilson’s tour de force
experiment in 1939(?): the radioactive Cl returned in the i-cholesteryl rearrangement without exchange with external normal Cl-. That’s a long way
to go along the edge of a molecule.
The retention/inversion selection must also depend on proton transfers. The nucleophilic water that attacks the hidden carbocation with inversion can either retain its protons and hence suffer subsequent displacement by a water on the same side as the departed chloride, or it could loose a proton by a chain mechanism (2-3 bridging waters) to the chloride, making it more difficult to eliminate. The first option would in effect give double inversion= retention, the second inversion. So it all seems to depend on the balance between the rate of “water-water” exchange vs the rate of proton transfer along a chain. Both would be expected to be fast.
I did once try to see if I could locate a [H3O]+ [OH]- ion pair in water. Each of the ions was solvated by 3-4 explicit waters (all in simulated water continuum). I tried both a contact geometry for the ion pair, and also one with 1 or more intervening neutral water molecules (solvent separated ion pair). All attempts at locating either type of ion pair failed because they were all quenched by (barrierless?) proton transfers.
So this simple classical mechanism of physical organic chemistry is far from trivial to study.