The so-called Fine tuned model of the universe asserts that any small change in several of the dimensionless fundamental physical constants would make the universe radically different (and hence one in which life as we know it could not exist). I suggest here that there may be molecules which epitomize the same principle in chemistry. Consider for example dimethyl formamide. The NMR spectra of this molecule reveal that at room temperature, the two methyl groups are inequivalent, indicating that the rate constant for rotation about the C-N bond has a very particular range of values at the temperatures at which most living organisms exist on our planet.
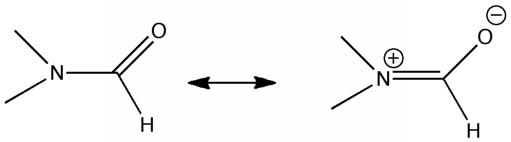
Dimethyl formamide
The half-restricted room-temperature rotation about the C-N bond arises from exactly the right amount of resonance contribution from the ionic form shown on the right, and this in turn depends on the relative energies of the nitrogen pair and the π system of the carbonyl group having the correct relationship. It is probably also true that the environment that this grouping finds itself in will alter the contribution (i.e. stabilize the ionic form over the neutral one). A little less contribution and the C-N bond would rotate much more easily, a little more and it would be much more rigid. Since this peptide bond is an essential and repeated feature of the structure of most biological proteins and enzymes, one might speculate that if that bond could rotate more easily, most enzymes would be much floppier than they are, and may not be easily induced to fold in a repeatable manner into the conformations that enable all the metabolic processes and make them the efficient catalysts they are. If the bond rotated less easily, it might be that the same enzymes would end up being too rigid, and this may prevent them from flexing sufficiently to allow key metabolites to enter or leave the active site.
Nowadays, the flexing of proteins is commonly studied using techniques of molecular dynamics, the driving forces for which are specified using molecule mechanics force fields. Here, the rotation about the C-N bond is defined by simple mechanical force constants or torsional barriers. I ask here how sensitive the dynamics of protein folding and catalysis are to the C-N rotational barrier? Is this truly a fine-tuned molecule, or might it be that the existence of life as we know it has a wide tolerance to the strength of the C-N bond?
Tags: active site, Interesting chemistry
Hi! I’m Diego and am a graduate student in Argentina.
You said…
“If the bond rotated less easily, it might be that the same enzymes would end up being too rigid, and this may prevent them from flexing sufficiently to allow key metabolites to enter or leave the active site.”
At a specific temperature, I think. Maybe if torsions where more rigid, life would develop at higher temperatures. Conversely if proteins where floppier, optimum temperature would be lower. Just a thought.
I’m just a newbe in MD, but maybe you could explore this using some MD package, like say, NAMD (which is free) or AMBER (which is not) changing parameters for torsions (or something like that, I’m just beginning with this).
Great blog!
[…] And Pauling took no account of any entropy differences between 2 and 3. In retrospect, 3 was simply too rigid to allow most enzyme catalysis models to function, as we recognise them […]
[…] resulting from the large dipoles in peptides (not least due to the zwitterionic character), the planarity of the peptide bond itself, the potential for other types of hydrogen bond (e.g. C-H…O) and entropic effects. I […]
I posted the above some four years ago. It is interesting to look at what modifications to the basic structures of life are made to allow organisms to survive in extreme conditions. One for example is the modification to survive at temperatures > 100°C, which in part involve using the Z-form of DNA (left handed helix) rather than the more common right handed forms.
Here is an interesting one just published, doi: 10.1073/pnas.1322003111 being a modification to enable survival at extreme pressures. At high pressures, proteins need stabilisation to allow for the osmotic pressures, and trimethylamine N-oxide is used in nature to ensure that the proteins are both stabilised and that their ability to fold correctly is not lost (a chemical chaperone). Thus fish that live at depths of ~ 7000m have the highest recorded cellular concentrations of trimethylamine N-oxide, and extrapolation of the osmotic properties suggests the maximum depth for fish to exist is ~8000m.
Quite how trimethylamine N-oxide manages to stabilise proteins in a molecular sense I do not know.