In an earlier post I wrote about the iconic SN1 solvolysis reaction, and presented a model for the transition state involving 13 water molecules. Here, I follow this up with an improved molecule containing 16 water molecules, and how the barrier for this model compares with experiment. This latter is nicely summarized in the following article: Solvolysis of t-butyl chloride in water-rich methanol + water mixtures, which (for pure water) cites the following activation parameters
- ΔH†283 = 23.0 kcal/mol
- ΔG†283 = 19.7 kcal/mol
- ΔS†283 = +11.1 cal/mol/K
But first, a word about how this new transtion state has been obtained. The DFT treatment used is quite standard (B3LYP/6-31G(d) ), and one can indeed locate a transition state using just this approach (this is how the previous model was obtained). One has to work very hard to orient the starting guess for the geometry so that as many hydrogen bonds between the waters themselves, and to the substrate, are created. The previous model took quite a few guesses and attempts! The solvent in such a model is simulated by the explicit water molecules themselves. Of course, the quality of the solvent then depends on how many water molecules are used. A proper solvent field using explicit water molecules is thought to require 100s of water molecules! But a reasonable approximation/compromise may well be 13.
So how can the model be improved? Well, in many ways, some of which include treating the dynamics of the system. But I will stick just to two.
- Firstly, we assume that the water molecules are used to form a bridge between the incoming nucleophile (another water) and the leaving group (the chloride). In the previous model, two such bridges were constructed using the 13 water molecules. But in fact, there is still space between two of the methyl groups to construct a third bridge. This takes the total solvent molecules to 16.
- Solvent can also be modelled as a continuum, in which a cavity which the substrate occupies is surrounded by a field generated by the continuum solvent. The problem with these cavity approaches in the past has been that it is not easy to optimize the geometry of the molecule contained within the cavity. Because the cavity was constructed by tesselation, the first derivatives of the energy of the molecule within the cavity were not regular, and as a result, geometry optimization (and particularly transition state optimization) would frequently meander and fail to converge. Darrin York and Martin Karplus came to the rescue (some time ago, it has to be said, DOI: 10.1021/jp992097l) by formulating a smoothed out solvation cavity where the first (and second derivatives) are stable and well behaved. This new algorithm has now been implemented in Gaussian09, and it now allows really easy transition state location within a solvent cavity
The result of this optimization is shown below (and can be seen in original form at the following DOI: 10042/to-2894).
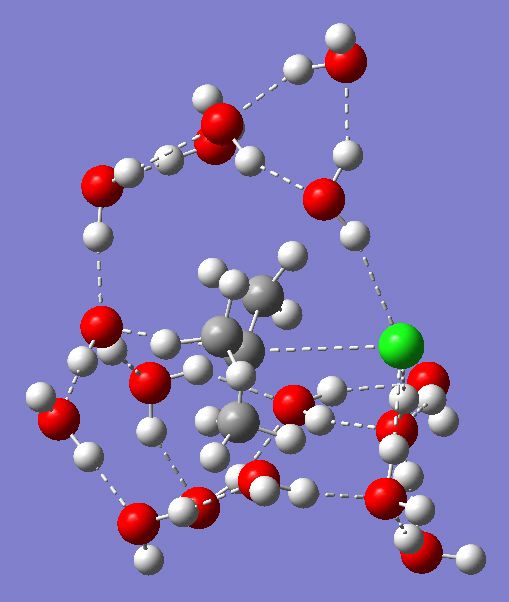
Transition state for Sn1 solvolysis of tert-butyl chloride. Click for animation.
- ΔH†298 = 17.4 kcal/mol
- ΔG†298 = 18.7 kcal/mol
- ΔS†298 = -4.4 cal/mol/K
The overall free energy is in great agreement with experiment! But the entropy is the wrong sign!! The calculation is predicting that the transition state is more rigid than the reactant. One can see how this might happen, since the greater ionic character produces very much stronger hydrogen bonds, which strengthen the three solvent bridges. It may be simply that the rigid-rotor-harmonic-oscillator approximation breaks down horribly for the entropy in this calculation. But it is encouraging that the activation barrier is reproducing experiment, which suggests the model cannot be completely wrong!
Tags: animation, Darrin York, energy, Historical, Interesting chemistry, Martin Karplus, Organic reaction mechanism, overall free energy
Most of the starting configurations had more waters around the chlorine, but since there was no confining box used, they wandered away (this is not a statistically tested assertion of course). Steve is of course correct that as there are more and more configurations, the entropy has to be handled statistically for the ensemble. This probably is a major cause of the error.
As to the mechanism, yes, the transition state can evolve dynamically in two directions:
Firstly, the initial trajectory can complete, in which case inversion has occurred. The original nucleophilic water than looses a proton by proton transfer along one of the water chains. Result: inversion.
Or, if the proton is not lost, a water molecule then attacks from the face previously occupied by chlorine, displacing the protonated water (easily the best leaving group) on the other face. Overall, retention has occurred via a double inversion.
So the reaction is pretty much defined by the dynamics of what happens around the transition state, and in effect which order things happen. With tert-butyl chloride, these two dynamic processes must be more or less equal, hence racemization.
Boy – lot’s of interesting things in this post! Here are a few comments:
1) We have done some microsolvation studies of amino acids (glycine and cysteine) and found that as one places ever more water molecules about the structure, there are ever more close lying configurations. So I do wonder whether the TS you’ve found is (a) the lowest possible and (b) whether a whole lot of other TSs are very close in energy. This will certainly affect the entropy. Given that the chloride carries the most dense region of negative charge, I would wonder whether there are configurations with more waters associated around the chlorine – both with just 16 waters, and especially when more waters are included.
2) But be that as it may, the TS structure has really got me thinking about just what species really exist in this solution. We teach the SN1 reaction as having in this case free t-butyl cation in solution. But what this TS suggests is that we get direct water displacement of chloride, from the backside, and then perhaps water-water exchange and that leads to racemic product? Does “free tbutyl cation” exist in aqueous solution?
Revising my statistical mechanics of course reminds of the simple formula that if there are N (more or less equal) microstates, the entropic correction for the ensemble is simply R*Ln N.
Working backwards, and assuming that the discrepancy between calculated and observed entropy is due to N equivalent microstates, one finds N ~ 2300. Seems a tad high to me, but it might account for some of the difference between calculated and observed entropy. Also one must consider the reactant configurations as well as those of the transition state, since its the difference between the two that controls the actual kinetics.
[…] For this example (see digital repository), the C-Cl bond length lengthens even further to 2.90Å which interestingly, is the same value it shows for the SN1 transition state! […]
[…] the motion is of mostly the two bromines and the central carbon. With the Sn1 mechanism, it is rotation of a methyl group (which is attempting to align with the carbocation centre being formed). This methyl motion is why […]
[…] the title hints, I have been here before. The SN1 solvolysis mechanism of t-butyl chloride was central to the flourishing of physical […]
This reminds me of an article by Johannes Dale in the Journal of Chemical Education 1998, 75, 1482 entitled “Inadequacies of the SN1 Mechanism” where he points out (in the conclusion) the following:
“I advised the students not to believe in the reality of free carbocation intermediates … [in aqueous solution] … … Many years later, these students remember my advice, but confess that they never believed me, since my view was not in agreement with the printed word of the textbooks.”
The article can be found here: http://pubs.acs.org/doi/abs/10.1021/ed075p1482
Look@s